022 - Combustion, fluid mechanics and fire safety engineering with Michael Gollner
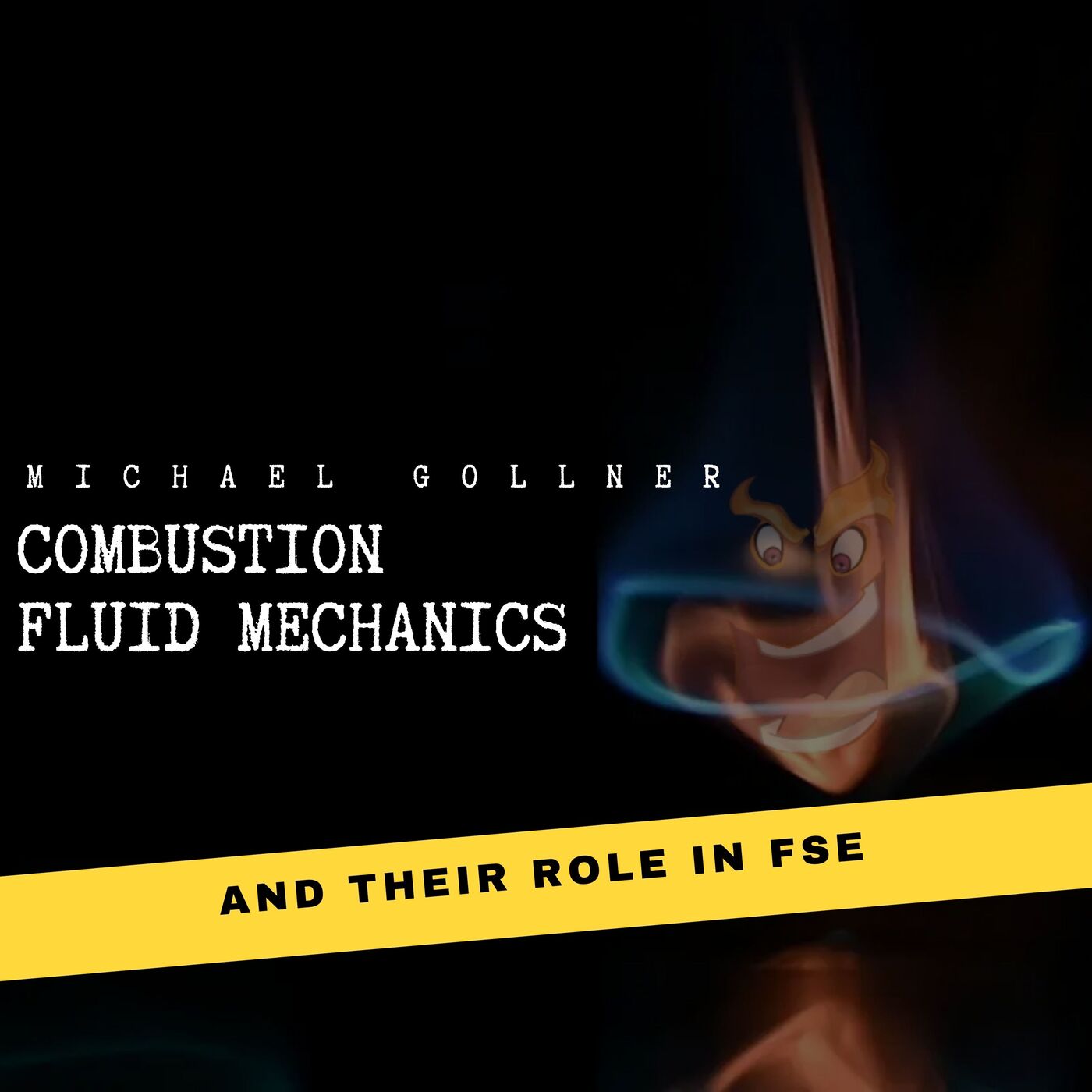
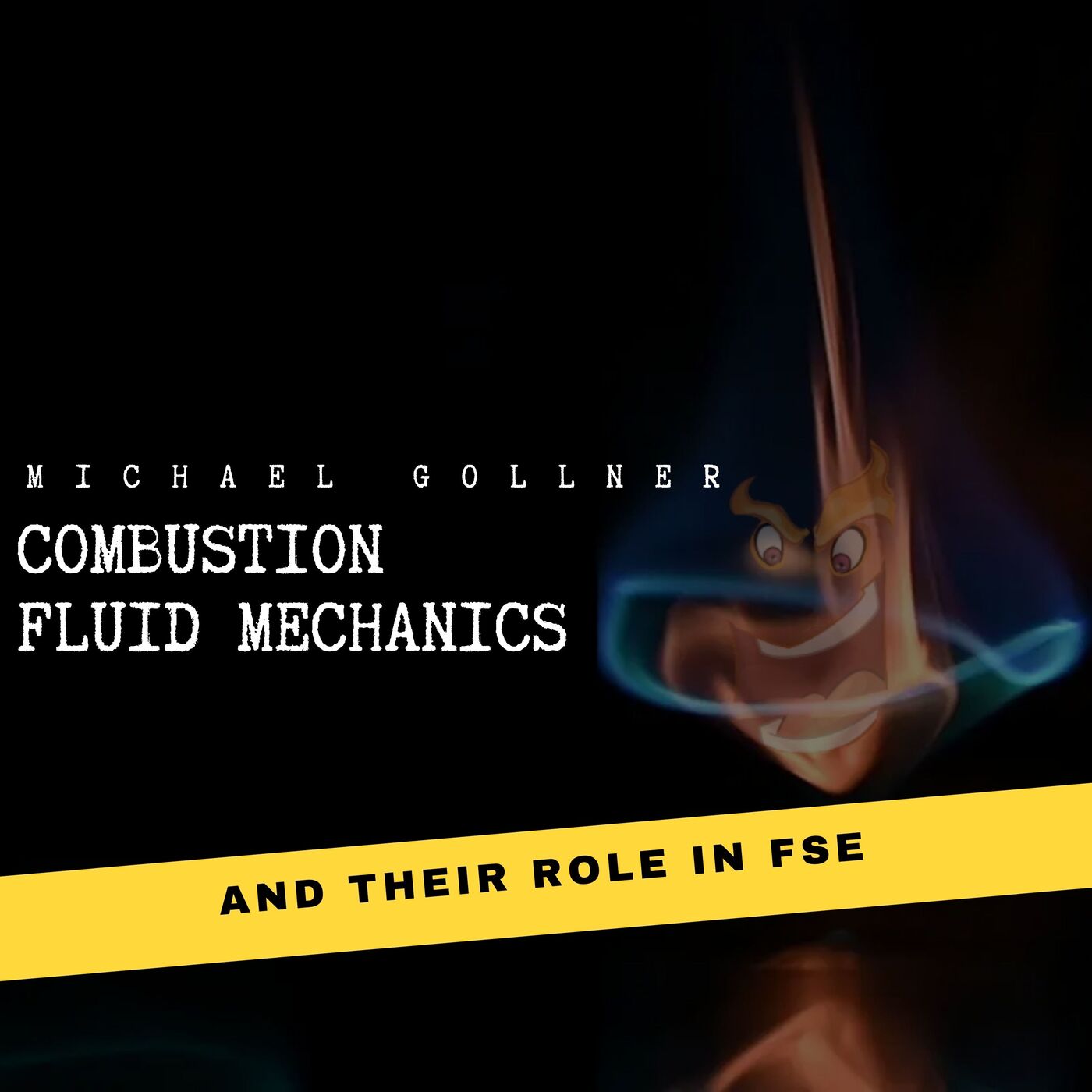
https://firelab.berkeley.edu/ this is the place you need to go!
Ignition at different slope angles. Firebrand spotting. Fire whirls. What does connect these various fire phenomena? They are all driven by fluid dynamics and can happen only in very particular flow conditions. To define and understand these conditions... well that is a bit longer story that I will unravel with Prof. Michael J. Gollner of the University of California, Berkeley.
In Episode 14 we have gone on a journey through scales of the fire phenomena with Sara McAllister (USDA). Today's episode is in a way follow up to that journey, as with Michael we try to understand why slope angle is such an important variable in the ignition of solids (and why peakHRR is not necessarily at the same slope as peak fire spread!). We venture into wind-driven crown fires and ember generation within them. Finally, we discuss the fire whirls, and notably - Michaels team discovery of "blue fire whirl" during his time in UMD. Although the topics seem very fundamental, we stay in touch with engineering... In the end, the influence of slope angle on the flame spread is interesting, and at the same time fundamental to predicting wildfire spread. Firebrand aerodynamics is fascinating, but if we don't understand that, we won't be able to build homes resilient to wildfires. Blue whirl is beautiful, but one day it may be the answer to efficient cleaning of oil spills. This episode is full of fantastic fire science, for everyone!
Connect with Michael on Twitter and LinkedIn
Check the collection of resources for fire protection engineers on Firelab Berkeley website.
Read up on Michaels research:
- Studies on upward flame spread (PhD Thesis at UCSD)
- M Finney et. al. Role of buoyant flame dynamics in wildfire spread PNAS
- A Tohidi, MJ Gollner, H Xiao, Flame Whirls Annual Review of Fluid Mechanics
- H Xiao, MJ Gollner, ES Oran, From fire whirls to blue whirls and combustion with reduced pollution PNAS
Hello, and welcome to session 22 of the Fire Science Show. First thing first, thank you to all of you supporting Fire Science Show through a five-star ratings, reviews, sharing the episodes with your friends or donating to the podcast or the podcast website. I really appreciate all the community support and I'm really happy that there is a community around this show that's rising up. And, that makes me very, very happy. Today I have for you another interesting guest. You maybe remember episode14 where I hosted Sarah McAllister and we went on a journey through the scales of fire phenomena. Let's say today's episode is a sequel to that. I am having a brilliant professor from University of California, Berkeley, where he has just achieved his tenure promotion. Today with me is professor Mike Gollner and we are gonna talk about combustion again, but again, we're not going to focus on a single phenomenon, but rather we're gonna unravel how fluid mechanics plays a role in fundamental combustion. What fundamental combustion is to fire safety engineering. And what interesting outcomes come from this to every engineer. So , I really hope you will enjoy this episode and yeah, let's not prolong this anymore. Let's spin the intro and jump into the episode. Hello. Welcome to fire science show I'm today with Professor Michael Gollner from University of California, Berkeley. Hi, Michael. Nice to see you.
Michael Gollner:Hey, great to be here.
Wojciech Wegrzynski:Yeah, so cool that you've joined the show and I'm really happy that it's just a few days after you've been promoted to your tenure track in Berkeley. That's amazing, man. Huge. Congratulations.
Michael Gollner:Thank you.
Wojciech Wegrzynski:I hope I'm the first one interviewing you as a new professor.
Michael Gollner:I think you are. I haven't done any interviews since, so I, uh, I feel honored.
Wojciech Wegrzynski:Yeah, man. The next step, the Noble Prize for your parking spots. But so far it looks promising. Let's see Michael, I've invited you because you are, quite familiar with combustion. I had in the show, Sarah McAllister who's taken me into fantastic journey through the scales of fire phenomena. And she's a person who is living from burning up, wood cribs and then I thought, you're a guy who's living from burning up a PMMA slabs. So that's pretty close. But, I think this, combustion topics are really fantastic because there is not that much exposure to fire professionals on fundamental combustion. At least there was not that much in my education and that's what I would like to discuss However, before we start, I'm actually curious. How much of fundamental combustion or fire phenomena you are teaching to your students who are pursuing like a master's in mech engineering
Michael Gollner:Well, it really depends. So as you know, I was a faculty in University of Maryland and there, you know, we had graduate courses in. Called diffusion flames, but in combustion. And so master's and PhD focused on that. And undergrad, there was certainly quite a bit of combustion in the fire dynamics. And here at Berkeley, there are no fire classes. Hopefully next semester I'll get to teach the first. So there is a wildfire taught in sort of the environmental science and policy side, but hopefully I'll get to teach the first engineering wildfire focus course. And the next semester to. And then we have traditional combustion, which used to be more energy and, you know, engine focused, but we're trying to transition this, my grad students, obviously that are doing research, get a little more into detail, but I'd say as well, that not, not all of my students are focused, on fundamental combustion. I mean, we have. It's such a mixed field, heat transfer, fluid mechanics, there's the combustion, but in wildfires, we're looking at risk analysis. We're looking at emissions and it's, our field is so interdisciplinary and tying things together. So yeah, within mechanical engineering, we do, we do a bit of the fundamental combustion, but I think it's a broader field and it takes a lot of perspectives put together.
Wojciech Wegrzynski:And if you get going to mind of like a graduate, a fire protection engineer, you think this topic would be very relevant to them? I'm honestly curious, because as I mentioned in my professional education in Poland, we had classes with late professor Konecki, my further supervisor who was teaching, compartment five dynamics, you know, Dougal Drysdale style compartment, fire dynamics, and that was huge fun. I really loved it, but, you know, premix flames, diffusion flames, not so much. And, then when. I'm a consultant. I'm a, let's say field engineer. I don't feel that I lack this knowledge, but every now and then I meet a problem, where without, this fundamental knowledge on, especially turbulent flames, it's difficult to solve the issue. And when I jumped into modeling and fluid mechanics is something that we both do. I don't need this knowledge to run the CFD because it's a black box. If I tell him to burn it will, but to understand what's happening in it, I. If I didn't get this knowledge elsewhere, I would have difficult times. So I wonder from your perspective in, graduate, fire safety engineer curriculum, how much should they know about, pretty fundamental combustion? Cause you like, look to me like a combustion person.
Michael Gollner:I looked like a yeah, well, you know, yeah. Well, this is always, this is the weird point tonight. I remember it. Uh, symposium professor Fernandez Pello who's here with me at Berkeley received the Emmons award, gave a lecture and was saying how he was everyone always thought in the combustion community. Oh, you're really in the fire community, the fire community, all you're really in the combustion community. And I feel like I, myself will always be in between. And now I have the wildfire and fluid,
Wojciech Wegrzynski:So you are in the triangle.
Michael Gollner:Although I take back, I said that everyone has been kind and accepting and everything. I don't feel like anyone, you know, but at the same time, everyone has this assumption. It's hard in an interdisciplinary field, but going back, you know, no, I don't think, an engineer needs to solve the partial differential equations so that he can formulate the Emmons problem. But you do have to understand what makes a fire burn. Okay. Where's the heat transfer coming? Why is the flame heating this up and making it spread? And what are the limits? Because you have your textbook common, you know, fire problems, and then you run into something new. You never want to be tricked into thinking something's just this common thing. If there's something unusual. So I'll take, I'm sure something we'll talk about inclined flame spread and the rate of burning and how something's happening, where the flame is based and fixed is different than what goes on up ahead. And in all the planes depends on the tilt of the flame and the ambient conditions. You don't need to be able to write the equations. I mean, in school, we're going to teach you the write the equations, but we want you to be able to think about it and to be able to understand that. And even for a firefighter, I think that intuitive knowledge is important. Cause you, you get a sense of what the fire might be able to do and how it's behaving, how it's going to change in different types of fuels, how the ventilation conditions. And so I would stress if you think of the mathy part. Oh, you know, let's make sure they know the boundary conditions. Let's make sure they know how the equation is going to change, even if you're not able to formulate it. Yes. I can think about it physically. And this is so important for modeling, like you mentioned, and that's, you know, this was the argument I made when I taught fluid mechanics to fire protection engineers. Why are we ever going to need this equation? I'm like, well, twenty-five years ago, practicing engineer. No, you're not going to need to do more than pipes for sprinklers, except yet, wait, you are, because you're going to run a CFD code and you put garbage into a black box. You get garbage out. How are you going to do grid? How are you going to run a CFD? If you don't understand, you know, the basics fluid mechanics and what goes into a Navier Stokes equation, do you need to solve it for a CFD code? No, but you need to know the pieces that work and what's assumed, and what's not because you know it, if you were installing a sprinkler system, if you don't think of the pressure at the head, I mean, you, you can arrive at so many. There's so many factors. And so I focus on that. I think people should have that fundamental understanding so they can think of what would happen, and be surprised when something unusual happens and try to figure it out. I think that kind of knowledge is important for, for all fields dealing with fire and let's face it fires kind of dangerous. It's, it's good to get an intuitive sense of what's happening.
Wojciech Wegrzynski:However, that intuitive sense is very difficult in fire. This is my theory. People think in a linear manner and fires are very non-linear and for example, we're in the commissioning a road tunnel now in Poland. And we were doing a test fire in the. in which we wanted to set he detector above where we were located with the fire. So we've done approximately one megawatt pool fire in it. And, everyone was, yeah. That's not big enough. That's really a small fire. We need a bigger fire to set that up. Okay. So we've done like 1.3 megawatt fire, a little bigger. We've merged the pans together. It was a bigger fire. They were like, no, no, no, this fire is not that big. So we've pushed it to the limit. And from our pans we've received like something, 1.7 megawatt fire. That's not a huge, change if you consider it's only 400 kilowatts more, but at this fire, everyone was like, oh my God, you're going to destroy the tunnel. That fire is too big... And like, no, no, it's just, it's the same incremental rise as the previous one it's, it's just, you know, radiation is in the fourth power of temperature. So if we change the temperature by a few hundred degrees, you are starting to feel it on your skin and this, if you don't know the fundamentals, if you don't recognize the fact , radiation is the fourth power of temperature or that the plume flow is in the 3/2 to power of heat release rate. You lose the ability because he will think that things change linearly and they are not. And the second story, when I discovered the importance of combustion and that segways to the inclined fires. I, approach the project in my career where we wanted to, determine the consequences of the fire of a large screen that was hanged underneath the sports arena roof. You know, like these huge boxes they have in the middle of sports arenas. So I wanted to figure out what's the size of the fire of that thing. And there was absolutely no resources that would allow me, but it's made of like simple plastics ABS or something. So I figured out, okay, let's calculate it. And it's okay. I didn't, we made up the number at the end. I was not able to calculate that. So here, this inclined fire spread, if you are able to solve that, that's brilliant. And that's something you've done in your PhD, right? You are testing inclination aspect on the flame spread that. Tell me
Michael Gollner:Careful how you stated, what would you state solved it? Oh, not at all. We found new nuances. No, I mean, the point we came up with was that the burning rate in this pyrolysis region, varies differently than the heating downstream. And so as you incline the fuel, it may spread fastest at a different angle than which it burns the fastest. So the heat release rate and the spread rate aren't necessarily correlated. And to me, this goes back to flammability. How do you classify flammability? Flammability now has to take into account geometry, which we always knew it did for the thickness of a material, but you incline it differently. The fire may spread differently under earth gravity. Let's not even get into microgravity
Wojciech Wegrzynski:No, no, no,
Michael Gollner:We can get there later, but you have to consider that the behavior of how the flame is centered over there and how the heat's transferred, which has something to do with radiation and the shape of the flame. And then how it's transferred downstream, how the plume attaches and how that changes, where the angle, those two mechanisms sometimes are aligned of when they increase and other times they, they deviate. And so you can have flames that spread really fast, but don't have a high burning rate. And then another time, you know, you can have the pool fire with a high burning rate, but almost no spread rate because just the angle and the radiation. And so. You know, if you're saying flammability, it's very hard to say, do you mean the ignitability? Do you mean the potential heat release rate? Do you mean the maximum potential spread rate? Because sometimes the material, all of those are high. Sometimes only one of them is. And so I think it just goes back to what I was saying for combustion. You don't want to think of just an outcome and that's why I sometimes hate pass, fail tests. I always like to think.
Wojciech Wegrzynski:I'm doing them for a living.
Michael Gollner:I know, and, and we work with them too, but you know, you want to, you want to get a number and you want to know why you have it so that you can really classify. I mean, there has to be a line drawn. That makes sense. I mean, you have to choose a line, that works, but you also have to think that there's a reason why something is behaving worse or not. That allows you to improve. And that allows you to think of, well, in a real situation, that's this test actually testing reality or not, you know? So is this material going to be flammable in the situation that I'm applying it? Is it right next to a heat source? Is there something different? So that's why we see so much work in fire because that isn't always taken into consideration. And even though a material or something passed the test, it then fails and we have a lot of work.
Wojciech Wegrzynski:You've mentioned the pyrolysis zone and because I'm prepared. I know there's a pyrolysis zone, a flame zone and plume zone. And, but I would love if you could, tell the listeners how these zones, differ from each other and. Like what's driving the phenomenon in each. What differs and where is the boundary between them. Because you said that the spread may be not the same thing as the, let's say, peak heat release rate, or you may have quicker spread or maybe larger heat release rate, but not necessarily at the same angle. So where would be that, boundary that differentiates between the spread and the maximum heat release rate? That's like, wow, that's fascinating.
Michael Gollner:Yeah. So when we talk about this, we're thinking about pyrolysis. Pyrolysis is just the burning material coming out. So as we know, you know, it's, let's say we have a piece of plastic, so it's the solid plastic stuff that's burning, but it's not burning while it's a solid it's, being vaporized. So you're getting pyrolysis gases as we call it. So flammable vapors are coming out from the heat, from the fire or the surroundings, and those are igniting. And then we have what we call a diffusion flame. Oxygen, the fuel they meet together. they burn, it's not like your stove, which is premixed and beautiful blue. Instead we get our sooty yellow flame with it's not perfect, but the fuel and oxygen meats. So let's imagine it's just this flat piece of plastic. You get the fire burning on top. You have that nice yellow flame, that whole region of the plastic burning. That's your pyrolysis zone. That's the area that's actually. Now, if you take that, the flame goes up, we call that a pool fire, right? The flame cause rising up. And there's all beautiful correlations for how high that plume might be. Now, if you tilt that and you put it vertically up, part of that is still that pyrolysis zone that's burning. And some of that flame goes up. Buoyancy is creating a flow in driving that flame up. But now that flame is no longer pointing straight in the air. All along that surface, cause that surface is a vertical piece of plastic. And so all of that zone ahead of that burning region is getting hot, right? That flame is adding a lot of heat to the unburned, or virgin materials as it's often called in the literature. So as that material heats up, it's eventually going to get. And that's going to cause flame spread. So flame spread. It's just this process. You have the burning, depending on the geometry and the conditions, the flame has heat transferred to stuff that hasn't burned. Eventually the stuff that hasn't burned ignites and it spreads and it can be constant. So in like what we call thermally thin. So like a piece of paper, it may burn sort of constant. So the same amount of stuff is burning because it burns out really quick and a thick piece of plastic. The plastic just keeps going. And so that material can just accelerate because more and more stuff is burning over time. And a lot of times in like a wildfire, it is acceleratory over a slope because more and more materials burning as it goes up. And so even though we talk about solving for how fast the fire may spread, that number is just some estimate because really it's changing constantly over. Because more and more things are being ignited. So those are sort of the stages when we think about it and then the heating in any zone, they're not necessarily coupled. So the heating to the stuff that's burning when it's a pool fire, you've got a big flame on top. And if it's radiation dominated, yeah. The bigger, the flame, the radiation is going straight down into the pool. And so it's getting a lot of heat there. Now we tilt it vertically and the area above that's now getting a lot of heat from the flame, but the flame has stretched. So now there's less flame heating the burning region, but more heating the spreading region. And so there, you kind of have this balance of some regions might have faster spread, but the burning rate starts to go down because the flame isn't painting it as well and vice versa. And it's not just by angle. It depends on how big it is, the bigger the flame, the more radiation, less convection. So these factors all play with each other.
Wojciech Wegrzynski:This theater of different heat transfer modes is fascinating. And. To my, fellow engineer practitioners. This is so, more practical than you would think, because if you consider, for example, a facade fire, that's exactly what Michael described. It's a thin flame on the surface of a vertical body. And even if your facade let's say is incombustible, You still have this heat transfer to the facade, which, let's say is aimed at destruction. It will cause some mechanical failure of the elements sooner or later. But now we, as the fire scientists or fire engineers were often, and I had a podcast episode about it. We often focused on measuring temperatures. So if you pluck a thin thermocouple into your inclined fire, into your pool fire. The number will be roughly the same, probably even higher in your vertical fire because of the better better diffusion. Right. But if you would calculate the heat transfer during the second of the burning to your surface element, that's next to that. It could be much less. And, it's, as engineers, we often focus so much on temperatures and the temperature in my, on my wall at the flame was like, 500 degrees. That's horrible. But you never take into account that it was. Five centimeter thin flame, which has a radiation factor of like, oh, 0.1 or something, because it's very thin. So it doesn't really matter that temperature was that high. And, only when you, are aware of this phenomenon, you are able to actually quantify the threats, right?
Michael Gollner:Right. And, and this isn't just in the fire protection field. I mean, in wildfire, which I do a lot of work and, and, you know, caring California, there's an awful lot, you know, this temperature was much hotter. was it really hotter?
Wojciech Wegrzynski:It was higher than melting point of steel.
Michael Gollner:Yes, but it's, it's all about the radiation and it's all about, I mean, it convection too, but it's about the heat flux. I'm teaching heat transfer to mechanical engineers this semester. And so explaining the importance of heat flux is, is a major goal of the class because temperature is going to be important if you're worried about the steel object and whether it starts to degrade or the glass, and it's going to shatter, or whether you're at the point of ignition of a material, because the thermal theory works pretty well. But if you want to understand the heating and whether something will ignite, it's got to be heat flux, which is the rate of heating, which causes that temperature rise. And so. Most people in the, in the public don't understand that nuance. Like you said, it's critical to actually getting a fundamental understanding. And I was actually, before you said it, facade fires are just a perfect example of where this comes to play and not just the facade. So you could have flames coming out of a window up ahead. You could have it burning over these flammable substrates they put on, but, I think it was a student of Jose Torero when I first started at Maryland visited, and was working before everything was really well known about these facade fire issues, where they were looking at that flow in gaps. So what if you had a gap between two materials? Why would we have a cavity flammable facades, right. You fill it with expandable foam, the foam. And now the heat's reradiated and the foam burns out super quickly and it drips. And so, even though it passed the test, when you specially sealed it, when you actually put it on a building, there's so many fire safety hazards there,
Wojciech Wegrzynski:Because scale matters. It's a different animal. When you put it on a 10 meter, long wall, three meters tall, then a small sample in your facilities. And also if you make a facade test and you are lucky with the placing your cavity barriers just below the thermal couple levels. Well, congratulations. You've passed the test, but, . Nope. It's difficult for me to do these statements because I know it's ridiculous. And I run the fire lab, so it's, I hope I don't get fired for this, but
Michael Gollner:No. I mean, but this is what I'm saying. I mean, we need tests. We need to define a line between where we consider acceptable. Nothing's a hundred percent safe. It's never going to be, but what's that acceptable level that we want to strive for where we can mitigate it. The people at the test labs, right. And the people designing that's the job of the engineer to think about these issues and to make sure that that. Judiciously choosing a test and judiciously designing the material. And if you think through the process of what a fire may do, you're doing some real due diligence. I think of. I'm trying to design a safe system. There's always unexpected, but we, you know, that's we keep learning. That's why we listen. There's the research codes get updated. And, but that's why I love tests that don't just give a pass fail. But if we learn more, we might learn that we have to change that threshold. We have to modify and allows us to look back.
Wojciech Wegrzynski:Thats one of the things that, is carried through the podcast for a long time. That observation is surprisingly powerful and maybe sometimes we should be more focused on seeing and understanding our experiments rather than putting a thousand thermocouples inside and just creating an un- uh-bearable plot of all of them. You've mentioned a few times the wildfires and the, theme close to your heart. You also said that you're in this triangle of wildfires science, combustion and fire safety engineering. That's that must be a fun position, actually. So for now in our discussion, we had the inclined fuel. We had, my huge screen or a bunch of plastic materials and the against the flame. But in wildfire, it gets more complicated because you're playing with porous fuels. Probably changes a lot. At least Sarah told me does. How would the, this, inclination play a role in wildfire and maybe you can tell me, for example, in the crown fire, w what really is driving the fire and what an engineer should know to have this intuitive understanding of what the fire could look like.
Michael Gollner:Yeah. So, so I'll step back. Cause if we want to teach for an engineer. So I was really lucky to have the opportunity to teach wildfire to engineers in Maryland. And, you know, the first thing to understand is you lose control in a wildfire. And I say that because in a building, we designed a fire safe system. We estimate the fuel load. What fuels can you bring in? We have suppression systems. We have barrier walls in a wildfire. You don't the weather change. And every day the sun shines the fuel load grows, right? Like it's, it's continually growing and then there's like ecological changes and you say, oh, I'll add a firebreak. Well, firebreaks don't help very much. I mean, it's, it's more for firefighting and fire suppression, to give those those gaps, unless the fire is pretty small, which it is sometimes that doesn't help much. So basics of wildfire, right? It can spread on the ground such as surface fire. It can, you know, either the smoldering fire on the surface, it can spread through the surface fuels like the brush, the grass, and the trees. And then it can also jump up to the crowds which you mentioned. And so this crown fire is often considered extremely hazardous because the fire tends to race both because there's higher winds at the top of the crowns and there's less, drag and also because you've got incredibly loose fuels, think of those pine needles, which also have flammable fuels in them that we haven't fully understood Sarah McAllister, who is a previous guest, has looked into some of that, but it can spread incredibly rapidly through those, those. You know, loose fuels and it takes some extreme conditions. It has to be pretty dry. You have to have a fairly large fire that can jump up the snags and stuff into the crown, but when you get sustained crown fires, they do move very fast. And So, you know, in wildfire, we're often looking at spread rates and so crown fires are, are very fast. And I don't think they're fully understood either. Another situation is that fires can be driven by wind and fires can be driven by slope. And so the way it makes sense, you blow more wind the flame tilts ahead, heats more material faster and spreads faster. So long as the materials they're on a slope. The same thing happens except it's not like the wind forces. The fire pulls the wind and drives itself. And so there's a self acceleratory process. And some of it can happen on flat ground too, with a big fire driving its own weather, but oftentimes on a slope, that's just a natural process with a fire. The buoyancy is pulling more air, which pushes the flame down. And so it drives itself and, and it doesn't move in a linear speed. It accelerates. It's not just like a 1-D process either. So if we think of the flame on just like a flat plane spreading up, it's going to spread and it may accelerate. But what if you like curve in those walls and make it a ravine that sloped? Well now there's no air flowing in from the sides. And now that flame has nowhere really, as the air is pushing in to go, but just slam flat on this. And suddenly you're jetting hot flammable vapors far ahead of the flame front. And it's just going to cause this dramatic acceleration. And so it looks like Domingos Viegas in Portugal, and others have. Become a receptive fires or accelerating fires. You know, I think eruptive is probably the wrong term, but there are situations for the slope fire spread, where you can get very fast flame spread and it loops back to fire protection engineering. There were a lot of investigations regularly by Dougal, Drysdale after the Kings Cross fire in London. The King's cross fire occurred in the tube station, where they had an escalator, which was sloped up and the walls were all shellacked wood, which was very flammable. And, um, we still don't know if the, if there was a pressure movement of the tube moving through, but essentially the fire was forced through that tube to move up and it just spread incredibly rapidly. Cause that flame is just pushed against the flammable materials, igniting more and more and accelerate. That can happen to wildfires too, and have been the cause of some of these deadly burn overs because people don't expect it to just jump in the behavior.
Wojciech Wegrzynski:You're back to the intuitive understanding of a fire, because this is a particular combination of conditions in which this particular flaming behavior can occur. If you didn't have this valley through which the wind would flow, you would not have this behavior. If it was like free to ventilate through it, you would not have this behavior. If you didn't have the fuel prepared for burning, you will not have this behavior, but it's this extremes in which people lose lives, actually, and that's common in. not only wildfires, it's common in building fires and in building fires also, it's not a well for civilians, it's all in the fire growth where they usually, lose their life, being surprised by the fire and without the way of escape, but for firefighters. It's when the ventilation path changes. that's the difficult one. Someone opens the doors to the, backyard or something, at the lower level. And suddenly the pressure plane drops in the building and it's all different for the people inside. That's how many firefighters lost their lives. There are phenomena like backdraft that are also intimately connected with this, flow and fire theater and creating this explosive almost a combination of fuel and oxygen that promotes very rapid burning that in the seconds changes the scene. So in wildfires, this is also probably something very dangerous for the people on the ground
Michael Gollner:it's the changes. And so we it's often termed extreme fire behaviour and I think it was more recently, redefined as basically any fire behavior that's unexpected, it's considered extreme. And so I talked about the fire, but let's say, let me tell you where wildfires are really challenging embers or firebrands. So it's not just the fire that you're tackling, but the fire will send flammable pieces, high into the air. And shower them ahead of the fire at locations, unknown to start new fires. And this has also resulted not only in destruction of communities, but also in deaths of firefighters, because you don't expect a fire to pop up in front of you, and then it's behind you. And suddenly you have an entrapment situation. But there's, you know, a lot of research going on to these embers or firebrands, which are almost always smoldering. They can be flaming to. They're smoldering pieces. They have lofted in diploma. They, they can literally go miles or kilometers ahead of the fire front and start new fires. And so, that really changes the dynamic of how you think about it.
Wojciech Wegrzynski:Man. You're so good. You could run the podcasts on your own. That's the next point on my list. Spotting and firebrands. And I'm personally a huge fan of the work of Ali Tohidi the Nigel Kaye and, yourself. On the firebrand aspect of wildfires and the spotting phenomenon, I think it's absolutely fascinating. And it's, something that's really ties, the wildfires science to building science, because it's this little chunks of burning material that connect both worlds in many cases. Right. So, tell us a bit more about this, phenomenon. How does it come to life and how in the hell the fire is able to throw 20 centimeter chunk of wood over distance of nine kilometers because it's unbelievable.
Michael Gollner:Well, you have to see fires to understand it. So, um, you mentioned Ali Tohidi, who was a postdoc of mine, and now is a professor, at San Jose state also here in the bay area in California, uh, Nigel Kaye and Clemson university. And NIST does a lot of work because there's a, there's a small, you know, clique who works in these embers and firebrands and, you know, any material as it burns out cellulosic or like, you know, woody material is going to start to char and it's going to smolder and think of those glowing embers, that charcoal. Just like your fire. You see the little sparks, the little things that are flying off. Those are embers. They're just small. Now think of a fire. That's blown pretty hard and you have all those little sticks. The pine needles themselves, they burn out pretty quick, but the sticks and the bark and the pieces and the wind's blowing as it chars. And as it degrades, it starts to kind of crumble and crack and things break off. And some of those things, if a fire has 200 foot high flames,
Wojciech Wegrzynski:Um,
Michael Gollner:don't know how to convert that into meters, but it's very large.
Wojciech Wegrzynski:very large.
Michael Gollner:And silently you can loft something. That's the size of a log or something it's rare, but it can happen depending on the wind speeds. And so what happens is that just a blizzard or a shower of these materials can fly ahead. If you have enough wind and a high enough plume, the fire itself is also pumping air up, right? It's buoyant and. It can pump embers up too. And so some of them can get lofted into the larger atmospheric flow and land up ahead. And that's most of the basis of our ember grid codes like that predict where they go or all about what's the maximum distance they can go. So how far can they go in the plume before they run out of fuel? Smaller anymore. There's a lot more that we try to do with that now. And it's not, you know, it's all sort of being added over time, but that was the basis of those Ember codes and how we calculate how far they could go. But that's what happens. Those sticks degrade. And we did some experiments at IBHS insurance Institute for business and home safety in South Carolina. And they have this giant, a wind tunnel that can blow down a house.
Wojciech Wegrzynski:me.
Michael Gollner:We were just burning, basically Christmas trees and they were running these experiments. We got to record them and you can see as the, as the fuel degrades, the fire finishes and it's smoldering. And so each stick just starts to like, it starts to bend a little bit and it flies off flies, it flies. And then you just get the shower from a single bush or a single tree and imagine a whole forest. Now, a lot of them just landed in. But a few may actually ignite and there's more closer to a fuel, but sometimes it can land far ahead. So it's so stochastic, it's so random, but so important.
Wojciech Wegrzynski:What's the lifetime of this particle when it lands, is it like in seconds, minutes hours?
Michael Gollner:Depends on the size of the, of the particle and the wind speed. It's encountering. I don't want to go too far into technical detail, but yeah, it just, it, it depends on how much fuel is in there. Remember smoldering, if it's not flaming is a slow process, so it can fester and smolder for, for a good period of time. It could, it could be an hour scale.
Wojciech Wegrzynski:Because if it's not in seconds, but more in like minutes or hours, it means these things can accumulate over time. And probably with every, another ember that is trapped at a certain location of your building, you are creating this higher probability that they will transition to flaming and then create,
Michael Gollner:Yes.
Wojciech Wegrzynski:an ignition conditions for your building.
Michael Gollner:And you said the next factor, right? So I was just talking about how they get generated and how they get lofted, but then eventually they ignite things. And so there's a lot of experiments, whether we like pile them up in beds,of pine needles.. If you get enough in a bed of pine needles and some wind, poof, they're always going to ignite in a bed of dry pine needles, but on a structure, it's pretty rare to get a single ember to ignite. But if you get a couple or you get a pile, then it, starts to become more and more likely that your deck or, or something in a crevice is going to ignite. And. It's igniting is this interesting process. Cause you have a smoldering piece which maybe doesn't have quite enough energy and then you have more smoldering pieces added. And eventually that has enough heat to start to smolder the material lands on. And then enough wind picks up where it transitions from smoldering to flaming, which to make our lives harder is again, a very stochastic and completely. It's not completely random, but it's all probabilistic. You know, any experiment has to be rerun them a bunch of times, because one time it'll ignite the next time. It won't. So we would say at a diets, maybe 60% after doing 30 experiments, you know, we, you know, transition to, flamings a really hard thing to characterize, but that's critical to whether you're going to start a new spot fire or you're going to ignite that part of a building and, Yeah, I think there's been some nice experiments by, uh, Sam Manzello and Sayaka Suzuki in Japan and that NIST where they have this dragon. So they throw a lot of embers at a structure and you can see them accumulate and then eventually ignite and, and understanding that process helps us think about how we design.
Wojciech Wegrzynski:Fascinating because if it was not hard enough, you're now creating an aerodynamics problem of building in winds and where the under pressure zone will go. When the, where the vertices will form around the building and our buildings are not. Aerodynamic. They're very, vortice friendly in terms of where these things, can land and yeah. I saw pictures from, I think it was the Bay Area where you were hit quite hard with wildfires people with these huge logs after, after a wildfire, was really an astounding that The fire can spread like this. But then again I guess most of us don't appreciate the size of a large wildfire, like really massive wildfire.
Michael Gollner:Yeah, I mean, Berkeley here has, has a long history, the tunnel fire in 1991, which Pat Paganini, who was. The leader in the fire safety community for many years, investigated. And they found that a lot of the spread was driven by Cedar shingles. So if you're not familiar, they're very light, lightweight, very, we've tried them in the lab super easy to ignite and they break into little like before. Which just they're perfectly aerodynamic. And so they're just going to float down the hill to the next one and, and they will, they won't even necessarily be smoldering. They'll retain the flame pretty well. And so it's a worst case scenario of something that's easy to ignite and flying the fire to your neighbor's house. And that was a really deadly incident just due to the timing. It wasn't huge as a fire. It was just, the conditions and the smoke people couldn't get out.
Wojciech Wegrzynski:It's not always the big fires that are the worst and it's not all deadly fires that were big. It's another thing that goes into the intuitive, seeing of the fires, not necessarily, it doesn't have to be big on my list. I have one. Final thing. And that's the, probably the coolest of them all now, or maybe hottest of them all, actually it's the fire whirls. For some reason I didn't understand, you've made a scientific career on fire whirls. They are super fascinating, super interesting. And they come in so many different, let's say flavors. And I really enjoyed her review on whirl fires. And they range from tiny ones to ones that devours cities. But there's this one that, you have observed as the first or your group has observed as the first, the blue firewood. And I remember it was like a, it was really cool for the community because it was something from our community that went viral and it was everywhere. Everyone was talking about your blue whirls please tell me the story, how you guys, have observed this for the first time. And what was your reaction to this discovery?
Michael Gollner:Okay, well, this, this is a fun story. So, I think. Most people know I was working on flame spread and things. And so a new colleague Elena Rohn, who's very well known and numerical combustion explosions. High-speed so not fire at all. And we were chatting about things we'd like to do. And we also had some discussions with, Lex Smits another professor at Princeton who was playing with firewhirls. Because he liked them. And he was from Australia originally and wanted to learn more. And we said, well, how can we work on this? You know, it's a fire safety issue in wildfires, but like tornadoes, you're not going to put them out. You're not going to, I mean, we already have an idea, you know, Saito and its others have a good grasp of where they can form, but what do we do from here? And then we were with one of her post-docs , and one of my grad students J Singh watching YouTube videos, literally. And we saw a fire world form and a Jim beam, factory fire. So this is a whiskey plant and it turns out we did some discovery channel special later. It turns out when we investigated this, the original distilled. So they actually diluted before. They give it to you. So before they do that, it's very high alcohol content and lightning struck this plant and the lake formed of it and the giant fire and a fire whirl spun up. And when we looked at it, we saw, wow. It almost looks like it's eating that and then somehow we got into the Deep Water Horizon and we're looking at how they were cleaning up oil spills within C2 burns and something clicked. And we said, oh, maybe we can clean up oil spills with this. And that's what started it. We took a disaster and said, how could we use this phenomenon for good? And let's be honest. How could we get funding to do this work? We didn't get much. Okay. We stretched it thin as much as we can. I had a grad student. iram who was amazing and use the support to do some amazing work on this. But what happened was a late night with, with a Wawa who's a postdoc and is now a professor at USC and, Jay who's now a professor in , and they were playing with this. And, we were like, well, let's, let's keep optimizing the fire whirl until we can get like, really clean, like how good can.
Wojciech Wegrzynski:Yeah.
Michael Gollner:And then one time the fire whirl just went whoop and became this little, perfectly blue spinning top on heptane. I mean, it's like gasoline, it doesn't burn blue, it doesn't get premixed on itself. And so a lot of people have said, oh, we've observed that before as it goes down. But I think something that changed was the fact that we did it over water. It was perfectly smooth. And the walls just. So the way I make a firewhirl in the lab is the walls are tangential. So like the air comes in at opposite directions. So it has to spin inside that chamber. And so just tweaking it over a couple of weeks, came up with. Now as a caveat soon after, uh, there's a little mishap, some crude oil spilled on the side, lit the experiment on fire. The fire department came, there was some delays. So always be luckily we had some very skilled people and, the fire extinguisher, we were provided didn't work. So they had to run to get another one, which is why the smoke detector trip. Then it's a long story. We have designed it. So that, that can't happen now. But. Another reason to think about what could happen in any experiment. But the discovery of the blue world was, was a fascinating and fun thing. And I think the encouragement, uh, that Elena, Ron, my colleague there gave for us, let's just dive into this new discovery rather than just going down the path of just characterizing. Let's just let's dig into this. This is so interesting. And that's what we did. And we were able to publish it and understand. And we've gone back. We're working on cleaning up oil spills with these larger firewalls as well. And we've seen significant reductions. And so when we formed them over crude oil fires, but, the blue whirl was this discovery that we've missed something and a behavior that we've been seeing because we, we changed the focus. We changed the approach, down into it was going it's fun.
Wojciech Wegrzynski:That's a, that's fascinating in a way, understanding physics of this, fire behavior is directly pathway through technology because you mentioned that it's, it can be used to clean oil, but it's also a very pure combustion when it's blue. And If we can get the less messy combustion, it provides us the ability to do things in, let's say more, environmental friendly way, maybe not in terms of CO2, but in terms of every other nasty thing that we emit while we are burning stuff. So. This is definitely much, much appreciated. And, as I mentioned, the whirls go into different flavors. There's also these huge worlds that happen in wildfires or even city conflagrations is this something also that, um, was of interest to your group or?
Michael Gollner:Well, I mean, in some ways, so you, you mentioned, so we had this review paper, which we, we wrote for, Annual Review of Fluid Mechanics. So at a very fluid mechanics flavor of, of what, what processes were driving the creation and how did they form it? You know, fire whirls form at all scales, we see the little blue whirl and the centimeter scale. I don't know if it can go much smaller. We haven't seen that yet. All the way up to kilometer scale in, atmospheric flows and, colleague, Neil and, University of Nevada, Reno is now doing more and more studies of these larger scale fire whirls that are at an atmospheric scale. They're literally tornadic and there hasn't been some devastating effects for them, but I feel like they're being seen more and more in fires as we get more of these extreme fires. You know, fire whirls are going to form, it's sort of this vortex phenomena. Whenever you have the right conditions, you need that upward source of buoyancy, which is going to come from any fire and you need something to generate that swirl. Could be the trees. It couldn't be the wind. It can be the topography. So they often form on the backside of a slope on the edge of a fire as it's spreading the winds, right. It'll spin them up and they kind of like run along the ends. And then if the whole shape of the fire is right and the wind is right, you can get these very large structures, that form, which is sort of what they think happened. Like after the Tokyo. The Great Kanto Earthquake Fire, which was maybe 30,000 people dead or something terrible like that. We still don't understand everything about them, despite what we've done, which is fascinating. But I think a lot of the mechanisms that started and probably similar, but as we saw from the blue whirl there's a lot of nuances in that in each process. And so. Are we going to stop all the fire whirls by understanding it? No, but we can still learn from it and learn where we might predict where there there's a possibility. I mean, these larger scale ones, they see signs of it on radar. I mean, like this is,
Wojciech Wegrzynski:You can see this whirly motion on the re wow.
Michael Gollner:Yeah. I mean, they see the same sort of signatures they look for for tornadoes. I mean, they're at an atmospheric scale and are they, we can fight over terminology. Are they still fire whirls if they comes from the, you know, fire weather from the ground up.
Wojciech Wegrzynski:They come out of your department into the tornado department not allowed to play with them anymore. That's that? That's so cool. Ah, man, the fire sciences is a fascinating place. To wrap it up, there's a, it's been an incredible journey from, inclined surfaces to crown fires, spotting to fire whirls and there's so much more to learn, on each of these, phenomena. And I usually ask people to refer to some resources, but actually, no, you have created an astounding base of resources at your Berkeley sites. So I probably link that even before my description of an episode. That's how good this site is. Here you have an airtime, advertise it as much as you want, and I will do more because it's amazing.
Michael Gollner:Feel free the firelab.Berkeley.edu. A lot of resources are thanks to postdocs and students, Franz Richter. Who's a post-doc came from Guillermo Rein's group where he's got his PhD prepared a lot for new, people interested in careers in fire science. So, yeah, please check it out. We post all of our papers too, and, I'm just, you know, happy to share it. I think it's really exciting to be working on the interface between the combustion and physical science of fire and how we apply it. And so that's also why we want to share the work is because we don't just want to do some esoteric, fundamental work, but we want to figure out what the real problems are, understand them and find the solutions and, and share them back with the community. That's our goal.
Wojciech Wegrzynski:And I'm very thankful for creating this a bunch of resources. I'm an user of them. I really appreciate, putting them all in one place. And it's really great initiative and they're absolutely worth checking as the second best place after the podcast to learn about fire science.
Michael Gollner:Definitely the Fire Science Show, first I, I I'm delighted that this, this came together. It's so nice to hear colleagues, especially in this time we can't travel as much, but hopefully these will be around for posterity. You know, it's just, uh, to hear people's perspective on the researchers is rare. And, and I, I appreciate it, as well as learning about other fields and how people are applying in industry, you know, we learn a lot.
Wojciech Wegrzynski:And the whole diversity of the fire sciences is just astounding. Okay. Michael, it was a huge pleasure to have you on the podcast. All the resources that we've talked about, the papers with discussed will be linked in the show notes. Thank you so much again for being here and yeah. See you the next time.
Michael Gollner:Yeah. See you the next time.
Wojciech Wegrzynski:And that's it. I hope you enjoyed that one. I really liked talking to Michael. He was on the list of my dream guests for the podcast for a very, very long time. Pretty much since I've started the show, I always wanted to talk to him and. I admire him a lot. He has a great career path in fire and definitely one of them young rising stars of our community. And I hope you understand why. His thoughts are really insightful and his research is really impactful on the world of fire safety engineering wildfires, and then fundamental combustion as well. For me, it was interesting to see how the fluid mechanics into play with fundamental combustion and how. All of this in the end is a flow phenomenon, a heat transfer phenomenon, and trying to find a way how to connect this with, with the real-world engineering. And I think we found some really good examples in the show that really illustrate of how important this fundamental aspects of fire science are for everyday practitioneer. And now for the end, I would like to highlight once more firelab.berkeley.edu. It's a brilliant web page filled with resources. I'm truly amazed how they've pulled it up together with Franz Richter And it's definitely worth checking out it's full of really useful stuff. So it really makes writing my show notes easier for this episode because everything is already out there. Anyway. Thank you so much for listening to this episode. I'm hope you liked it as usual. Please share it with a friend and share the knowledge about the podcast. Next week another great guest, another great episode. So yeah. See you next Wednesday. Cheers.